Prosthetic Solutions
The most basic prosthetic feet come in two types: Solid Ankle Cushioned Heel (SACH) and Elastic Keel configurations. These designs consist of crepe neoprene or urethane foam molded over an inner keel and shaped to resemble a human foot. Because they have no hinged parts, these basic feet are relatively inexpensive, durable and virtually maintenance-free. These feet offer cushioning and energy absorption but do not store and return the same amount of energy as dynamic-response feet. SACH and elastic keel feet are generally prescribed for amputees who do a limited amount of walking with little variation in speed.
SACH Foot: The SACH is the simplest type of non-articulated foot. The name refers to a somewhat soft rubber heel wedge that mimics ankle action by compressing under load during the early part of the stance phase of walking. The keel is rigid, which provides midstance stability but little lateral movement. The SACH foot is available in various heel heights to match individual shoes with different heel heights.
Elastic (flexible) Keel Foot: This prosthetic foot allows motion similar to that of SACH feet. In addition, the forefoot is able to conform to uneven terrain but remains supportive and stable during standing and walking.
Single-Axis Foot: The articulated single axis foot contains an ankle joint that allows the foot to move up and down, enhancing knee stability. The more quickly the full sole of the foot is in contact with the ground, the more stable the prosthesis becomes. This is beneficial for users with higher levels of amputation (an amputation anywhere between the knee and hip). The wearer must actively control the prosthesis to prevent the knee from buckling, and the single-axis ankle/foot mechanism reduces the effort required to do so. Unfortunately, the single-axis ankle adds weight to the prosthesis, requires periodic servicing, and is slightly more expensive than the more basic SACH foot. A single-axis foot may be more appropriate for individuals where stability is a concern.
Multi-Axis Foot: Although similar to the single-axis foot in terms of weight, durability and cost, the multi-axis foot conforms better to uneven surfaces. In addition to the up and down mobility of the single-axis foot, a multi-axis foot can also move from side to side. Since the added ankle motion absorbs some of the stresses of walking, this helps protect both the skin and the prosthesis from wear and tear.
People with more active lifestyles typically prefer a more responsive foot. A dynamic-response foot is ideal for those individuals who can vary walking speed, change directions quickly or walk long distances. Dynamic-response feet store and release energy during the walking cycle by absorbing energy in the keel during the “roll-over” phase and then springing back to provide a subjective sense of push-off for the wearer. Additionally, they provide a more normal range of motion and a more symmetric gait. Some dynamic-response feet feature a split-toe design that further increases stability by mimicking the inversion/eversion movements of the human ankle and foot.
The comfort and responsiveness of a dynamic-response foot can also encourage an individual to advance from a more moderate activity level to a higher activity level, given the more natural feel of walking with this type of prosthetic foot. Further, some dynamic-response feet have been shown to reduce impact forces and stress upon the sound side foot and leg.
Microprocessor-controlled (MPC) feet are a fairly new category of prosthetic components. These foot/ankle components have small computer-controlled sensors that process information from both the individual’s limb and the surrounding environment to adjust to various needs. Based on information from input signals, these processors apply an algorithm, or set of rules, to make decisions about how the ankle or foot should respond in any given situation. The microprocessor provides instructions to various parts of the prosthesis in order to produce the desired function of the foot. Current MPC ankles use a variety of sensors, including ankle angle sensors, accelerometers, gyroscopes and torque sensors. The microprocessors in these systems then take the input signals and make decisions as to how to position the ankle, how to set the damping resistance in the ankle, and how to drive an ankle motor during stance phase (1).
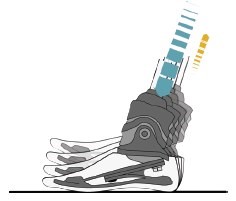
Figure 1: Relief Function of a Microprocessor Foot (source: oandp.org)
The largest potential benefit of an MPC ankle/foot system over other prosthetic feet is the enhanced ability to react to varying environmental situations by providing different mechanical properties or alignments to improve the user’s balance and mobility. For example, non-MPC prosthetic feet work nicely on smooth, level terrain; however, they have a more limited ability to alter their mechanical properties or alignment when walking on slopes or other uneven surfaces. Powered feet provide propulsion during ambulation to enhance walking capabilities in real-time. Some specific models include software as well as options for connectivity to mobile devices through smart phone or computer apps. This allows the prosthetist and user to match the performance of the ankle/foot to various activities, allow for adjustments to the input gains and timing, and turn on or off certain features. All of these functions provide a more individualized experience by the user.
The ultimate goal of this class of prosthetic feet is to mimic the functions of the human foot. However, devices differ in their ability to accommodate for all environments and thus to the extent in which that accommodation can be achieved (2). Although these types of feet can coordinate the movements of the foot and ankle automatically, they do not directly communicate with the body. Microprocessor or powered prosthetic feet require batteries to power the chip, sensors, motors and actuators. Additionally, electronic parts associated with microprocessor systems make them more delicate than their passive counterparts. Many should not be used in water or in highly dusty or dirty environments. Due to the extra parts required by the addition of the microprocessor, they often weigh more than other prosthetic feet. Users may notice the mechanical clicks and sounds coming from the prosthesis as the microprocessor extrapolates information and adjusts various aspects of the ankle or foot. Finally, the higher level of technology and more intricate design of this class of prosthetic feet mean they may likely be the more expensive options on the market.
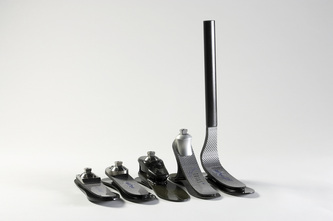
Prosthetic Knees
The single-axis knee, essentially a simple hinge, is generally considered the “workhorse” of the basic knee classes due to its relative simplicity, which makes it the most economical, most durable, and lightest option available.
Single-axis knees do have limitations, however. By virtue of their simplicity, the 09/10/2006control; amputees must use their own muscle power to keep them stable when standing.
To compensate for this, the single-axis knee often incorporates a constant friction control and a manual lock. The friction keeps the leg from swinging forward too quickly as it swings through to the next step.
Polycentric knees, also referred to as “fourbar” knees, are more complex in design and have multiple axes of rotation. Their versatility is the primary reason for their popularity. They can be set up to be very stable during early stance phase, yet easy to bend to initiate the swing phase or to sit down. Another popular feature of the knee’s design is that the leg’s overall length shortens when a step is initiated, reducing the risk of stumbling.
Polycentric knees are suitable for a wide range of amputees. Various versions are ideal for amputees who can’t walk securely with other knees, have knee disarticulation or bilateral leg amputations, or have long residual limbs.
A standard polycentric knee has a simple mechanical swing control that provides an optimal single walking speed; however, many polycentric knees incorporate fluid (pneumatic or hydraulic) swing control to permit variable walking speeds. The most common limitation of the polycentric design is that the range of motion about the knee may be restricted to some degree, though usually not enough to pose a significant problem. Polycentric knees are also heavier and contain parts that may need to be serviced or replaced more often than those of other types of prosthetic knees.
Some amputees need or desire the security of a knee joint that locks in extension to prevent buckling. One option is the manual locking knee, which incorporates an automatic lock that can be unlocked voluntarily. This is the most stable knee available. Walking is possible with the lock either engaged or disengaged, although the locked knee requires excessive energy to use and produces a stiff, awkward gait. The manual locking knee is appropriate for weak or unstable patients as well as more active individuals who frequently walk on unstable terrain.
Weight-activated stance-control knee. This knee is very stable and is often prescribed for a first prosthesis. When weight is placed on the prosthesis, the knee will not bend until the weight is displaced. This system functions as a constant-friction knee during leg swing but is held in extension by a braking mechanism as weight is applied during stance phase. This knee is a common choice for older or less active amputees.
All knee systems require some degree of swing control to maintain a consistent gait. In many cases, this control is provided by mechanical friction at the axis of rotation and is adjusted to match the normal cadence of the opposite leg. Constant-friction knee units are simple, lightweight and dependable. Their main disadvantage is that the knee is adjusted for a single walking speed at any given time.
Variable friction provides increased resistance as the knee bends from full extension. This provides “cadence response,” allowing variable walking speeds; however, this system requires frequent adjustment and replacement of moving parts and is considered less advanced than fluid control knee systems.
Advanced swing control for prosthetic knees uses fluid dynamics to provide variable resistance, enabling amputees to walk comfortably at different speeds.
These units consist of pistons inside cylinders containing air (pneumatic) or fluid (hydraulic). Pneumatic control compresses air as the knee is flexed, stores the energy, then returns the energy as the knee moves into extension. Gait control can be further enhanced with the addition of a spring coil. Pneumatic systems are generally considered to provide superior swing control to friction knees but to be less effective than hydraulic systems.
For active amputees, hydraulic systems provide the closest thing to normal knee function. Hydraulic systems use a liquid medium (usually silicone oil) instead of air to respond to a wide range of walking speeds. Although hydraulic knees provide a smoother gait, they are heavier, require more maintenance, and a higher initial cost.
Microprocessor knees are a relatively new development in prosthetic technology. Several such new knees are now available or in development.
Onboard sensors detect movement and timing and then adjust a fluid /air control cylinder accordingly. These microprocessor-controlled knees lower the amount of effort amputees must use to control their timing, resulting in a more natural gait.
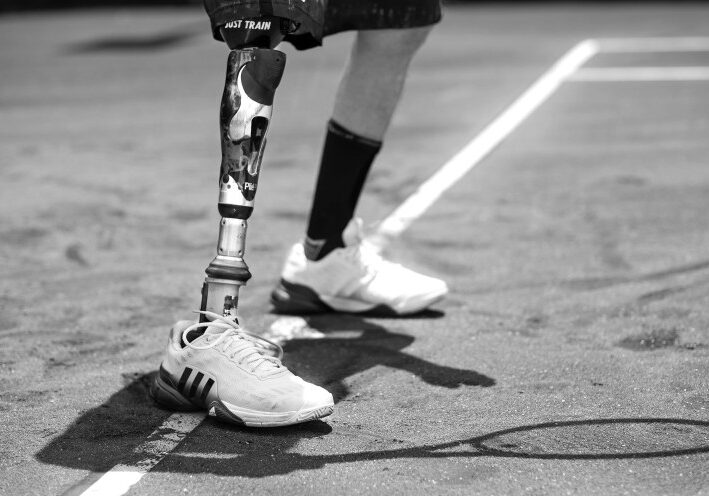
A sleeve creates a seal around the top edge of the socket, then a pump and exhaust valve remove virtually all air between the socket and the liner as you wear them. The system regulates the vacuum level within a defined range. Vacuum enhances how well your socket adheres to your limb, which reduces shear, regulates residual-limb volume changes and improves circulation in your limb.
In this case, you use a padded liner with a pin at the end. The pin is inserted into a shuttle lock built into the bottom of your socket, the only connection point. One variation for above-knee prostheses, called a lanyard system, uses a strap to pull the liner into the socket. The lanyard also connects the socket to the liner near the top, which slightly reduces rotation and shear.
A suction system consists of a soft liner, a one-way valve and a sealing sleeve. Inserting your liner-covered limb into the socket and applying body weight as you stand expels excess air through the valve. Suction provides even adhesion to the entire interior surface of the socket for security, stability and reduced friction and shear.
Shuttle-lock systems, best suited for older amputees and patients with reduced mobility, are mainly for amputees with a mobility or activity level of 1 or 2. Suction can be used for all activity levels. Vacuum is appropriate for activity levels 2 to 4, including the most active amputees
Vacuum and suction both require a total-surface-bearing socket, which distributes even pressure on your residual limb throughout every square inch of the socket’s surface area. That pressure relief alone makes these suspension systems more comfortable. The superior connection with vacuum, allowing minimal movement of the limb in the socket, makes it the most comfortable. Suction ranks second in linkage. Shuttle-lock systems offer the least control of forces and allow the most movement and rubbing in the socket, the cause of calluses, blisters and sores.
Vacuum offers the highest performance for maximum confidence and prosthetic control, which promotes a smoother, more symmetrical gait that uses less energy. Shuttle-lock systems fulfill the needs of users with a low activity level, but users with a high activity level probably would notice a lack of control and security.
Because a pump can generate five times the air-pressure differential of suction, vacuum scores highest here. Some patients say the sense of control is almost like having their leg back. Shuttle-lock systems have the lowest level of proprioception.
Vacuum excels at limb health. Vacuum actually increases hydration and blood flow, so open wounds can heal even while you continue wearing the prosthesis. In addition, vacuum helps regulate volume changes in your leg as tissues shrink or expand during the day. As a result, your limb retains its size and shape all day, which helps to maintain a consistent fit.
In contrast, other suspension systems constrict blood vessels, causing leg volume to drop an average of 10 to 14 percent as fluids are squeezed out of your leg throughout the day. A prosthesis that fits tightly in the morning will loosen as the day progresses. Too much movement within a socket can cause pain, blistering and sores. Movement in and out of the socket can lead to repetitive impact forces as the socket slides during while you’re lifting your leg to take a step and pounds it back onto your limb when you put weight on your foot again.
Shuttle-lock systems are the easiest to put on and take off. However, users of these systems may need to add or remove prosthetic socks up to three or four times a day to compensate for changes in leg volume.
With vacuum and suction, your daily routine includes rolling on a liner and shorter prosthetic sock; sliding into the socket; rubbing lotion on your leg at the top of the liner to prevent dragging on the skin; and rolling up the sleeve attached to the socket’s perimeter to create a seal with the liner.
Because there’s no need to modify the fit throughout the day, active vacuum may save on total time. Either way, most users say the morning regimen is worth it to enjoy the all-day benefits.